Cellular Innovations: Latest Research in Cell Biology
Cellular Innovations: Latest Research in Cell Biology
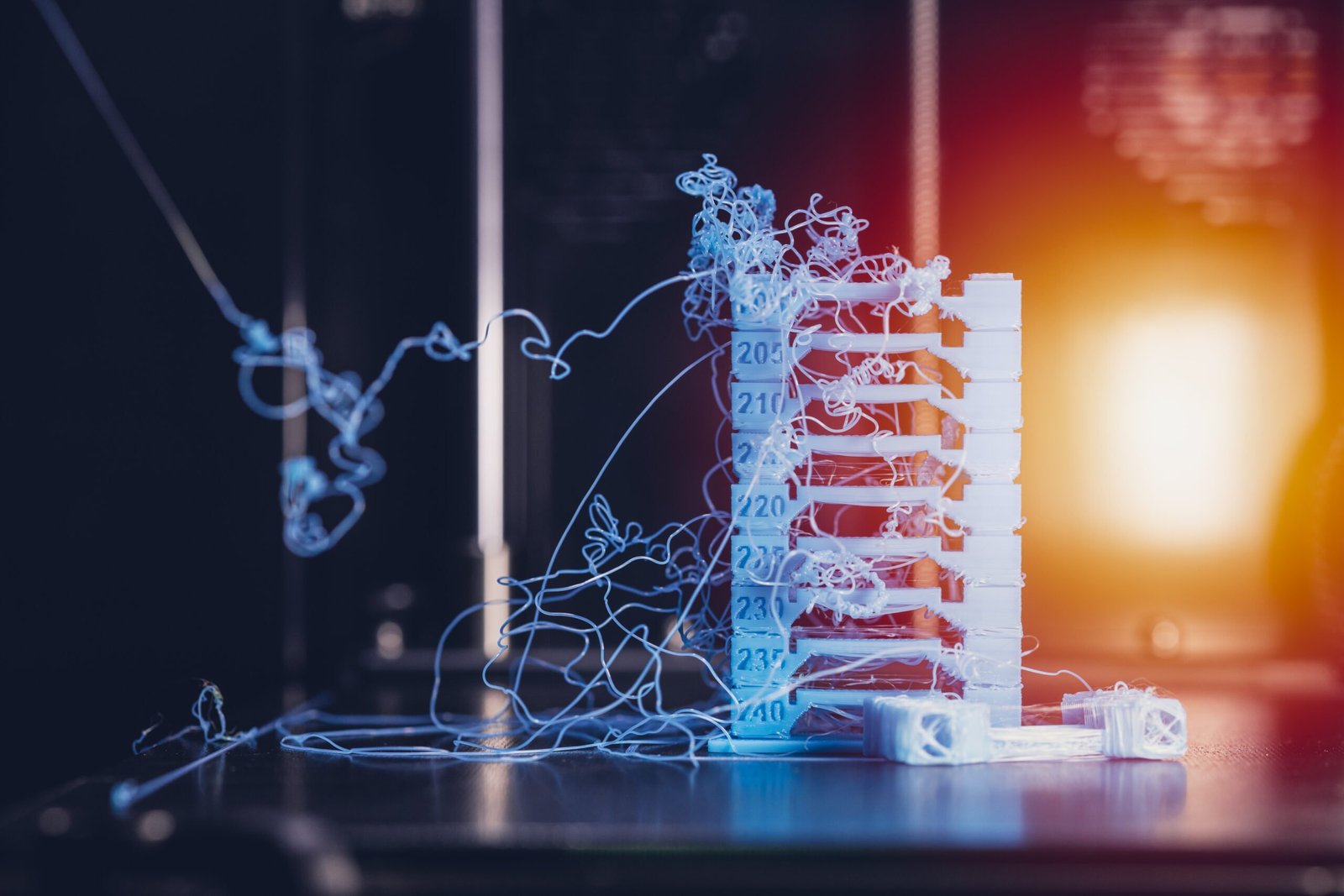
Introduction:
In the rapidly evolving field of cell biology, recent innovations are pushing the boundaries of our understanding of cellular mechanisms. From groundbreaking discoveries in stem cell research to the latest advancements in cellular therapies, scientists are unveiling remarkable insights that could transform medicine and biotechnology. This article delves into some of the most significant research areas in cell biology today, highlighting key developments, emerging trends, and potential implications for the future.
Stem Cell Research: New Horizons
Breakthroughs in Pluripotent Stem Cells
Pluripotent stem cells (PSCs) have the unique ability to differentiate into any cell type, offering immense potential for regenerative medicine. Recent studies have made significant strides in enhancing the efficiency and safety of induced pluripotent stem cells (iPSCs). For instance, researchers at Kyoto University have developed a new method that reduces the risk of genetic mutations, making iPSCs safer for clinical applications[^1^].
Furthermore, innovative techniques for generating PSCs are continually being refined. One notable development involves the use of CRISPR-Cas9 technology to precisely edit genes within stem cells, thereby improving their therapeutic potential. Researchers have successfully used this approach to correct genetic defects in iPSCs, paving the way for personalized medicine[^2^].
The potential applications of PSCs are vast, ranging from tissue engineering to drug testing. For example, scientists at Harvard University have utilized PSCs to create organoids—miniaturized and simplified versions of organs—that can be used to study disease mechanisms and screen new drugs more effectively[^3^].
Advances in Mesenchymal Stem Cells
Mesenchymal stem cells (MSCs) are another area of intense research due to their potential in regenerative therapies. Recent findings have shown that MSCs possess immunomodulatory properties, making them suitable for treating inflammatory diseases. Researchers at the Mayo Clinic have demonstrated that MSCs can be engineered to enhance their anti-inflammatory effects, offering new hope for conditions such as rheumatoid arthritis and Crohn’s disease[^4^].
Moreover, MSCs are being explored for their role in tissue repair and regeneration. In a landmark study, scientists at Stanford University successfully used MSCs to regenerate damaged heart tissue in animal models, showcasing their potential in cardiovascular medicine[^5^]. This could lead to breakthroughs in treating heart failure and other chronic heart conditions.
The versatility of MSCs extends to their ability to differentiate into various cell types, including bone, cartilage, and fat cells. This makes them a promising candidate for orthopedic and reconstructive surgeries. Ongoing clinical trials are investigating the effectiveness of MSC-based therapies for conditions like osteoarthritis and spinal cord injuries[^6^].
Cellular Therapies: Transforming Treatment Paradigms
CAR-T Cell Therapy
Chimeric Antigen Receptor T-cell (CAR-T) therapy represents a cutting-edge approach in cancer treatment. By genetically modifying a patient’s T-cells to target specific cancer cells, CAR-T therapy has shown remarkable efficacy in treating certain types of leukemia and lymphoma. Studies conducted by the University of Pennsylvania have reported high remission rates in patients with refractory B-cell lymphoma[^7^].
However, CAR-T therapy is not without challenges. The treatment can cause severe side effects, including cytokine release syndrome (CRS) and neurotoxicity. Researchers are actively investigating ways to mitigate these risks. For instance, scientists at Memorial Sloan Kettering Cancer Center are developing next-generation CAR-T cells with “safety switches” that can be activated to control adverse reactions[^8^].
Beyond hematological cancers, CAR-T therapy is being explored for solid tumors. Early-phase clinical trials are underway to evaluate its effectiveness in treating cancers such as glioblastoma, lung cancer, and ovarian cancer[^9^]. If successful, these efforts could significantly expand the therapeutic applications of CAR-T cells.
Gene Editing and Cell Therapy
Gene editing technologies like CRISPR-Cas9 are revolutionizing the field of cell therapy. By enabling precise modifications to the genetic code, these tools offer the potential to correct genetic disorders at their source. Researchers at MIT have successfully used CRISPR to edit genes in human hematopoietic stem cells, demonstrating the feasibility of this approach for treating blood disorders such as sickle cell anemia and beta-thalassemia[^10^].
Additionally, gene editing is being combined with cell therapy to enhance the effectiveness of treatments. For example, scientists at the University of California, Berkeley, have engineered T-cells with CRISPR to increase their ability to target cancer cells[^11^]. This combination therapy holds promise for developing more potent and durable cancer treatments.
Ethical considerations surrounding gene editing are also a critical aspect of ongoing research. Ensuring the safety and ethical use of these technologies is paramount, and regulatory frameworks are evolving to address these concerns. Collaborative efforts between scientists, ethicists, and policymakers are essential to navigate the complex landscape of gene editing in cell therapy[^12^].
Organoid Technology: Modeling Human Disease
Creation and Application of Organoids
Organoids have emerged as powerful tools for modeling human diseases and studying developmental biology. These 3D structures, derived from stem cells, mimic the architecture and function of real organs. Researchers at the Hubrecht Institute have pioneered techniques to grow organoids from various tissues, including the brain, liver, and intestines[^13^].
One of the key advantages of organoids is their ability to replicate human-specific disease processes. For instance, scientists at Johns Hopkins University have developed brain organoids to study neurological disorders such as Alzheimer’s disease and epilepsy[^14^]. These models provide valuable insights into disease mechanisms and enable the testing of potential therapeutic interventions.
Furthermore, organoids are being used to investigate infectious diseases. Researchers at the University of Cambridge have created lung organoids to study the effects of respiratory viruses, including SARS-CoV-2, the virus responsible for COVID-19[^15^]. These studies are crucial for understanding viral pathogenesis and developing effective treatments.
Drug Testing and Personalized Medicine
Organoids offer a promising platform for drug testing and personalized medicine. Traditional drug testing methods often rely on animal models, which may not accurately reflect human physiology. Organoids, on the other hand, provide a more relevant and scalable system for evaluating drug efficacy and toxicity[^16^].
For example, researchers at the Institute of Molecular Biotechnology in Vienna have used intestinal organoids to screen for drugs that could treat cystic fibrosis[^17^]. This approach has led to the identification of potential therapeutic compounds that are now being tested in clinical trials. Organoids can also be derived from patient-specific cells, enabling personalized drug screening and tailoring treatments to individual patients.
The integration of organoid technology with high-throughput screening methods is further accelerating drug discovery. Automated systems for generating and analyzing organoids are being developed to enhance efficiency and reproducibility. This convergence of technologies holds great promise for revolutionizing drug development and reducing the time and cost associated with bringing new therapies to market[^18^].
Cellular Communication and Signaling Pathways
Understanding Cell-Cell Interactions
Cellular communication is fundamental to the functioning of multicellular organisms. Advances in imaging and molecular biology techniques are shedding light on the intricate networks of cell-cell interactions. Researchers at the University of California, San Francisco, have employed advanced microscopy to visualize how immune cells communicate and coordinate their responses to infections[^19^].
One significant discovery in this area is the role of extracellular vesicles (EVs) in intercellular communication. EVs are small particles released by cells that carry proteins, lipids, and RNA, facilitating the exchange of information between cells. Studies have shown that EVs play crucial roles in processes such as immune regulation, cancer progression, and tissue repair[^20^].
Moreover, recent research has uncovered the importance of physical interactions between cells. For instance, scientists at the University of Cambridge have demonstrated how mechanical forces influence cell behavior and tissue development[^21^]. These findings are opening new avenues for understanding developmental biology and designing tissue engineering strategies.
Signaling Pathways in Disease
Disruptions in cellular signaling pathways are often implicated in diseases such as cancer, diabetes, and neurodegenerative disorders. Understanding these pathways is essential for developing targeted therapies. Researchers at the Broad Institute have mapped key signaling networks involved in cancer, identifying potential drug targets to inhibit tumor growth[^22^].
One major focus is the Notch signaling pathway, which regulates cell differentiation and proliferation. Dysregulation of Notch signaling has been linked to various cancers, including leukemia and breast cancer. Scientists are exploring inhibitors of Notch signaling as potential cancer therapies[^23^].
Another critical pathway is the PI3K-Akt-mTOR pathway, involved in cell growth and metabolism. Aberrations in this pathway are associated with cancers and metabolic disorders. Inhibitors targeting this pathway, such as PI3K inhibitors, are being developed and tested in clinical trials[^24^]. Understanding these complex signaling mechanisms is crucial for advancing precision medicine.
Conclusion:
The landscape of cell biology is being transformed by innovative research that is unlocking new knowledge and therapeutic possibilities. From the remarkable potential of stem cells and gene editing technologies to the transformative impact of CAR-T therapy and organoid models, advancements in cell biology are poised to revolutionize medicine and biotechnology. As we continue to unravel the complexities of cellular mechanisms and interactions, the future holds promising opportunities for addressing some of the most pressing health challenges of our time.
Key Takeaways:
- Pluripotent Stem Cells: Enhanced safety and efficiency through novel techniques, including CRISPR-Cas9.
- Mesenchymal Stem Cells: Promising applications in regenerative therapies and immunomodulation.
- CAR-T Cell Therapy: Significant success in hematological cancers with ongoing research in solid tumors.
- Gene Editing: Revolutionary potential in correcting genetic disorders and enhancing cell therapies.
- Organoids: Versatile tools for disease modeling, drug testing, and personalized medicine.
- Cellular Communication: Insights into cell-cell interactions and signaling pathways critical for understanding diseases.
FAQs
1. What are pluripotent stem cells?
Pluripotent stem cells (PSCs) are cells that have the ability to differentiate into any type of cell in the body. This makes them highly valuable for regenerative medicine and research.
2. How do CAR-T cells work?
CAR-T cells are genetically engineered T-cells that are modified to target and kill specific cancer cells. This involves extracting T-cells from a patient, altering them to express chimeric antigen receptors (CARs), and reinfusing them back into the patient.
3. What are organoids used for?
Organoids are used for modeling human diseases, studying organ development, drug testing, and personalized medicine. They provide a more accurate representation of human tissues compared to traditional cell cultures.
4. What is gene editing?
Gene editing involves making precise changes to the DNA sequence within a cell. Technologies like CRISPR-Cas9 allow researchers to add, remove, or alter DNA to study gene functions or correct genetic disorders.
5. What are mesenchymal stem cells?
Mesenchymal stem cells (MSCs) are multipotent cells that can differentiate into various cell types such as bone, cartilage, and fat cells. They are used in regenerative medicine and have immunomodulatory properties.
6. How are organoids created?
Organoids are created by culturing stem cells in a 3D environment that mimics the natural conditions of the human body. These stem cells then self-organize into structures resembling mini-organs.
7. What are the challenges of CAR-T therapy?
Challenges include severe side effects like cytokine release syndrome (CRS) and neurotoxicity. Researchers are working on next-generation CAR-T cells with safety mechanisms to mitigate these risks.
8. What role do extracellular vesicles play in cellular communication?
Extracellular vesicles (EVs) are small particles released by cells that carry proteins, lipids, and RNA. They facilitate intercellular communication and play roles in immune regulation, cancer progression, and tissue repair.
9. How can gene editing be combined with cell therapy?
Gene editing can enhance cell therapies by correcting genetic defects or improving the therapeutic properties of cells. For example, CRISPR can be used to engineer T-cells to better target cancer cells.
10. What are some key cellular signaling pathways involved in disease?
Notable pathways include the Notch signaling pathway, associated with cancer and cell differentiation, and the PI3K-Akt-mTOR pathway, linked to cell growth and metabolism. Understanding these pathways is crucial for developing targeted therapies.